A narrative review of the role of glucocorticoid receptors in prostate cancer: developments in last 5 years
Introduction
Prostate cancer is one of the 10 most common cancers globally and poses a great threat to human health. In men, it is the most frequently diagnosed cancer in 112 countries in 2020 (1). Androgen deprivation therapy is a systemic basic therapy for patients with advanced prostate cancer, and the basis of novel combination therapy. However, despite a median of 18–24 months of treatment, most patients will experience cancer progression into castration-resistant prostate cancer (CRPC) (2-4). The mechanisms involved in the development of CRPC are complex (5). Most studies have focused on androgen receptor (AR)-dependent mechanisms.
Studies based on whole-genome and targeted deoxyribonucleic acid (DNA)-sequencing technologies have shown that the AR genes are the most frequently rearranged genes in CRPC tumors, which promote the expression of AR-variants genes. Li et al. [2020] examined ribonucleic acid (RNA)-sequencing data from a cohort of 101 prostate cancer patients and pointed out the enhanced effect of tumor metastasis and the resistance of enzalutamide due to expression of a novel AR-variants gene, and found that AR expression was higher in the experimental group than other groups (6). On the other hand, some researchers also found that the upregulation of AR-variants 7 is associated with development of CRPC (7-9).
AR-independent pathways are considered another factor involved in the development of CRPC. Shorning et al. [2020] summarized the new mechanisms of development of CRPC based on the interplay between the PI3K-AKT-mTOR pathway and several key oncogenic signaling nodes (10). The expression of microRNAs (11,12) and defects in DNA damage repair (13,14) in CRPC have yet to be researched.
Recently, some researchers noted that glucocorticoid receptors (GRs) are involved (15-17) in the development of CRPC based on emerging evidence that the use of glucocorticoids may contribute to tumor progression in CRPC (18-20). Glucocorticoids are widely used for prostate cancer therapies. They are not only used to arrest the growth and slow the proliferation of tumor cells, they are also effectively used to alleviate symptoms caused by the treatment and tumors (21). However, the mechanisms of their actions on prostate cancer cells remain unclear. Research has shown that GR activation promotes cell proliferation by inhibiting apoptosis, which may confer resistance to anti-androgens (22,23). In this article, we summarized the published data and new ideas on the effectiveness of steroid hormones and glucocorticoid receptors in CRPC in the past five years based on another paper (24). We present the following article in accordance with the Narrative Review reporting checklist (available at https://tau.amegroups.com/article/view/10.21037/tau-22-501/rc).
Methods
We summarized the isoforms of GRs and the mechanisms involved in CRPC. An updated literature search was performed on the effectiveness of steroid hormones used in CRPC from the ClinicalTrials database (https://clinicaltrials.gov/), the National Center for Biotechnology Information database (https://www.ncbi.nlm.nih.gov/) and European Union Drug Regulating Authorities Clinical Trials database (https://eudract.ema.europa.eu/). The focus was on the timeframe from 2017 to 2022. The general terms searched were “glucocorticoids” [Mesh] AND “prostate cancer” in NCBI database, “prostate cancer” and “glucocorticoids” in ClinicalTrials database and EudraCT database. More specific search criteria were then used: Prednisolone/Prednisone/Dexamethasone AND “prostate cancer”/“CRPC”/“castration-resistant prostate cancer”. The status should be completed or trials with results. At least one primary or secondary outcome [prostate-specific antigen (PSA) response rate, progression-free survival (PFS) or overall survival (OS) and median time to PSA progression] according to studies should be included (See Table 1 and Table S1).
Table 1
Items | Specification |
---|---|
Date of search (specified to date, month, and year) | 2022.06.01 |
Databases and other sources searched | Clinical Trials database (https://clinicaltrials.gov/), the National Center for Biotechnology Information database and European Union Drug Regulating Authorities Clinical Trials database |
Search terms used (including MeSH and free text search terms and filters) | Search terms: “glucocorticoids” [MeSH] AND “prostate cancer” in NCBI database, “prostate cancer” and “glucocorticoids” in ClinicalTrials database and EudraCT database. More specific search criteria were then used: Prednisolone AND “prostate cancer”/Prednisone AND “prostate cancer”/Dexamethasone AND “prostate cancer” or AND “CRPC” or AND “castration-resistant prostate cancer”Filters: Status: Completed or Trials with results |
Timeframe | 2015.03–2022.01 |
Inclusion and exclusion criteria (study type, language restrictions, etc.) | Selection criteria: (I) results include PSA response rate, PFS or OS, median time to PSA progression as part of a study (at least one primary or secondary outcome according to studies); (II) mainly focus on the effectiveness of glucocorticoids; (III) ≥15 patients participated in studies |
Selection process (who conducted the selection, whether it was conducted independently, how consensus was obtained, etc.) | Studies selected by Zhou and Shi independently. Then studies were integrated |
Any additional considerations, if applicable | N/A |
CRPC, castration-resistant prostate cancer; PSA, prostate-specific antigen; PFS, progression-free survival; OS, overall survival; N/A, not applicable.
GR
Nuclear receptor superfamily 3, group C, member 1 (NR3C1) encodes GRs, is located on chromosome 5 (region 5q31.3) (25), and consists of 9 exons. The alternative splicing and translation of exons has mainly produced 2 isoforms of the NR3C1 gene (i.e., human GRα and human GRβ) (26,27). The classic receptor human GRα has 3 major different functional domains: the N-terminal or immunogenic domain, the DNA-binding domain, and the ligand-binding domain (LBD) (Figure 1) (28,29). GRα can bind to agonist ligands, and thus dissociate from the heat shock proteins and subsequently regulate target gene expression through the glucocorticoid-responsive elements (GREs) (28). Recently, a study showed that lung cancer metastasis-related protein 1 promotes the transference from advanced metastatic prostate cancer to CRPC by activating the GRα signaling pathway (30).
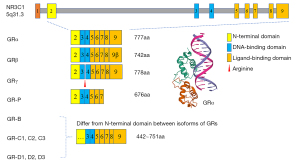
Another isoform of GR, named human GRβ, whose LBD is different than that of GRα, does not conventionally bind to glucocorticoid agonists. Recent researches revealed that GRβ is localized both in the cytoplasm and nucleus in a cell-type-specific manner (e.g., GRβ actions are restricted to its dominant-negative effects on GRα-mediated responses) (31,32). Notably, GRβ can bind to the glucocorticoid antagonist, RU-486 (means mifepristone) (33), which has an inhibitory effect on GRβ-coupled prostate cancer cell proliferation (34).
Similarly, GR and AR are both belong to the nuclear receptor superfamily, sharing the same chromatin binding domains (16,35). In some situations, the expression of GR may be upregulated during treatments of CRPC as well as parts of genes related in the AR-related signaling pathways. Puhr et al. (36) pointed out that GR expression is reduced in primary prostate cancer, restored in metastases, and correlates with reduced progression-free survival in relapse patients. Sahu et al. (37) found that the cistromes and transcription programs of GR and AR showed significant overlaps. Researchers exposed LNCaP-1F5 and VCaP cells to nonsteroidal antiandrogens and found half of the GR cistromes overlaps with the AR cistromes by ChIP-seq with the help of FoxA1 protein. Arora et al. (23) pointed out that the expression of GR was increased when AR was potentially inhibited by enzalutamide, which re-expressed about 50% of AR-responsive genes and promoted tumor proliferation. In other ways, Wu et al. (38) designed GR/AR dual antagonist based on the similarity in sequence and structure of GR and AR binding sites (hormone binding pocket). They predicted 10 compounds and picked out compound Z19. This compound directly binds to AR and GR and inhibits the proliferation of prostate tumor cells in vitro. However, a phase I/II trial (39) designed to apply dual AR-GR antagonism (Enzalutamide and Mifepristone) in CRPC patients reported that the addition of mifepristone to enzalutamide following a 12-week enzalutamide lead-in did not delay time to PSA, radiographic or clinical PFS.
Genomic and non-genomic effects of GRs
The effects of GRs are mediated by classical genomic and alternative non-genomic mechanisms. In classical genomic methods, the interaction between GRs with either GREs on the DNA or the negative GRE mediates the regulation of gene expression (40). Rapid non-genomic effects mainly include: (I) specific interactions with membrane-bound GRs; (II) non-specific interactions with plasma membranes; and (III) a specific interface with cytosolic GRs (41,42) which can affect mitochondrial function within seconds. However, an exploration of the non-genomic action of GRs in prostate cancer is still incomplete. An explanation for the development of androgen-dependent prostate cancer to hormone-independent prostate cancer is the point mutation of AR (43) and therefore the development of drug resistance. SGK1 is a known target gene induced by ARs and GRs, and plays an important role in GR-mediated CRPC progression (22). The inhibition of androgen-induced genes shows that GRs can regulate the expression of AR-target genes without ARs, which suggests that GRs can bypass the AR inhibition of drugs by regulating different but overlapping transcriptomes to make the tumor appear hormone-independent (23). This also suggests that there is a mechanism for tumor drug resistance; that is, increasing the expression of GRs.
Glucocorticoids as therapeutic agents
Glucocorticoids belong to the steroid family, mainly synthesized in the adrenal glands, which are released in response to stress and in a circadian way (44,45). Glucocorticoids are widely used in the treatment of inflammatory and autoimmune diseases and organ transplantation. It is worth noting that the dosage and usage of glucocorticoids should be strictly controlled because of their short-term or long-term side effects (46-48), such as hyperglycemia (49-51), adrenal insufficiency (52), osteoporosis (53), and Cushingoid features (54).
Glucocorticoids have played an important role in the treatment of leukemia and several solid tumors, relieving the discomfort of chemotherapy drugs in the past decades. During the treatment of childhood leukemia, glucocorticoids were used as the first choice, which may regulate the expression of a series of apoptosis-related genes (55). The death-associated protein named DAP acts as a positive mediator of programmed cell death that is induced by interferon-gamma. Hulkko et al. (56,57) reports that DAP3 interacted with GR mainly via the N-terminal region of DAP3, modulating the cytoplasmic GR-hsp90 complex, finally induced apoptosis. Wang et al. (58) found that mometasone furoate could inhibit proliferation and migration of acute leukemia cells via regulating PI3K signaling pathway.
In the treatment of prostate cancer, synthetic glucocorticoids are extensively used in practice as an integral part of regimens based on their anti-inflammatory, the suppression of adrenal androgen synthesis and other benefits (59-61). However, evidences showed that the use of glucocorticoids may contribute to tumor progression in CRPC (15-17) In a post hoc analysis of the COU-AA-301 trial (62), corticosteroid use at baseline was associated with a worse overall prognosis, but not with less response to abiraterone. Woods-Burnham et al. (63) reported that glucocorticoids could induce therapy resistance-related stress oncoproteins. Stress oncoproteins Clusterin (CLU) and Lens Epithelium-Derived Growth Factor p75 (LEDGF/p75) were upregulated in response to standard PCa therapies (64-67). Knocked-down of GR by short inhibitory RNAs (siRNAs) reduced two proteins. Possibly there are multiple putative GR binding sites within the LEDGF/p75 and CLU promoters via in-silico analysis. Ortiz-Hernandez et al. (68) demonstrated that a group of interactome (JPO2, MEN, MLL, IWS1, ASK1, and PogZ, as well as transcription factors c-MYC and HRP2) may interact with integrase binding domain of LEDGF/p75, contributing to the survival, clonogenicity, and tumorsphere formation of docetaxel-resistant prostate cancer cells. However, the lack of researches and clinical trials still brings difficulties to define what extent glucocorticoids and GR contribute to the chemotherapy resistance.
Clinical trials in last 5 years
Prednisolone, dexamethasone, and prednisone are the major glucocorticoids applied in prostate cancer treatment. Under the guidelines (69), abiraterone combined with prednisone is a first line treatment for advanced prostate cancer. Due to adverse drug reactions, dexamethasone may be used in other combination therapies, such as those with ketoconazole. In the last 5 years, clinical trials or retrospective studies have focused on novel drugs based on the mechanism of selective inhibition or the applications of combination therapies. We chose studies from the ClinicalTrial database and EudraCT database and some retrospective studies from the NCBI database published in the last 5 years. The selected studies explored the effect of dexamethasone (70-76), prednisone (77-93), and prednisolone (94) (see Table 2).
Table 2
Study | Dosage | N | PSA response rate (%) | PFS or OS* (month) | Median time to PSA progression (month) |
---|---|---|---|---|---|
Dexamethasone | |||||
NCT00427999 | 1 mg QD | 65 | 37.7 | ||
NCT01036594 | Hydrocortisone: 20 mg po QAM + 10 mg po QPM, dexamethasone: 0.5 mg po BID | 32 | 25.0# | ||
Mehra (70) | 0.5 mg po QD or prednisone 5 mg po BID | 75 | 31.6 | 25.6* | |
Tanaka (71) | 0.5 mg po BID | 75 | 76.8 | 12.0 | |
Calvani$ (72) | 1 mg po QD or prednisone 10 mg po QD | 37 | 51.0 | 11.0 | |
Nakai$ (73) | 0.5 mg po BID or prednisone 5 mg po BID | 82 | 8.6 | ||
Noguchi (74) | 1 mg po QD | 51 | 53.8–56.5 | 7.4–8.9 | |
Zhu$ (75) | 10 mg ivgtt ONCE | 41 | 35.0 | 17.0* | |
Roviello$ (76) | 0.5 mg po QD | 36 | 11.1 | 2.5 | |
Prednisone | |||||
NCT00642018 | 5 mg po BID | 149 | 56.1–56.9 | 8.6–9.0 | |
NCT01940276 | 5 mg po BID | 100 | 66.0–74.0 | 16.6–16.8 | |
NCT01666314 | 5 mg po BID | 137 | 17.0–50.0 | ||
NCT02217566 | 5 mg po BID | 46 | 57.5 | 29.6* | 7.3 |
NCT01695135 | 5 mg po BID | 214 | 54.5 | 5.6 | |
NCT01193257 (77) | 5 mg po BID | 1,099 | 24.9 | 8.3 | 5.5 |
NCT01084655 (78) | 5 mg po BID | 37 | 59.0 | 6.8 | |
NCT02097303 (79) | 5 mg po BID | 36 | |||
NCT01308580 (80) | 5 mg po BID | 1,200 | 29.5–42.9 | 2.9–3.5 | 5.7–6.8 |
NCT01637402 (81) | 5 mg po BID | 41 | 12.0 | ||
NCT01511536 (82) | 5 mg po BID | 37 | 46.2 | 6.9 | |
NCT01393730 (83) | 5 mg po QD | 40 | 60.0 | 5 | |
NCT01193244 (85) | 5 mg po BID | 1,560 | 24.6–42.6 | 13.8 | 5.59–8.3 |
NCT01308567 (86) | 5 mg po BID | 1,168 | 60.7–68.7 | 4.4–5.3 | 8.2–9.2 |
NCT01543776 (87) | 5 mg po BID | 72 | 50.0–58.0 | 8.6 | |
NCT01204710 (89) | 5 mg po BID or 5 mg po QD | 121 | 22.6 | 2.3 | |
NCT00887198 (90) | 5 mg po BID | 1,088 | 30.0–34.0* | 11.1 | |
NCT01718353 (91) | 5 mg po BID | 63 | 55.6 | 9.1 | |
van Dodewaard-de Jong (84) | 5 mg po BID | 88 | 56.5–61.9 | 9.8 | |
Yu (88) | 5 mg po BID | 74 | 24.0–47.0 | 13.9–17.9 | |
Posadas (92) | 5 mg po BID | 57 | 32.0 | ||
Attard (93) | 5 mg po BID or 5 mg po QD or 2.5 mg BID or dexamethasone 0.5mg QD | 164 | 60.0–88.1 | 12.8–26.6& | 4.83–18.56 |
Prednisolone | |||||
NCT01685983 | 5 mg po BID | 82 | 42.7 | 4.7 | |
NCT00268476 (94) | 5 mg po QD | 1974 | Not reached |
$, research studies marked “$” in the “Study” column indicates a retrospective study; #, values marked “#” in the “PSA response rate” column indicates a decline of PSA ≥30%; *, values marked “*” in the “PFS or OS” column indicates it is the value of OS. &, values marked “&” in the “PFS or OS” column indicates it is radiographic PFS. Some data in this study (NCT00268476) had not reached median time. PSA response rate: A decline of PSA ≥50%. CRPC, castration-resistant prostate cancer; PSA, prostate-specific antigen; PFS, progression free survival; OS, overall survival; po, oral; QD, quaque die; QAM, every morning; QPM, every evening; BID, twice daily; ivgtt, intravenous drip; ONCE, only once.
The PSA response rate of dexamethasone is approximately 11.1–76.8%, and prednisone and prednisolone have been reported to have PSA response rates of 17.0–88.1% and 42.7%, respectively. Research has shown that the median time to PSA progression of prednisone is 5.0–18.6 months, while that of dexamethasone is 8.6–12.0 months (see Table 2) on CRPC.
Dexamethasone is usually administered with endocrine therapy drugs or other anti-tumor drugs, such as docetaxel, ketoconazole, imatinib, peptide cancer vaccine, and cyclophosphamide. A phase II, single-center trial (NCT01036594) sought to determine whether the administration of ketoconazole/dexamethasone, after disease progression, with ketoconazole/hydrocortisone slows or reverses disease progression. The results suggested that the number of participants who experience a ≥30% decline in PSA after change from ketoconazole with hydrocortisone therapy to ketoconazole with dexamethasone therapy was 8 (25%). However, low patients’ number included in this clinical trial leaved a question open. Another retrospective study evaluated the safety and activity of a steroidal switch from prednisone to dexamethasone in patients with advanced, heavily pre-treated CRPC (76), and found that 11% of the patients (4/36) showed a PSA decrease (≥50%). Thus, switching medications could be an option for patients who have previously responded well to abiraterone acetate treatment. Interestingly, a randomized, open-label phase 2 study (93) designed for assessment of the safety of glucocorticoid regimens in combination with abiraterone acetate showed that patients (n=42/164) took 1,000 mg abiraterone acetate with 0.5 mg dexamethasone once daily orally (other three groups: 1,000 mg abiraterone acetate with 5 mg prednisone twice daily/5 mg prednisone once daily/2.5 mg prednisone twice daily) had highest PSA response rate (88.1%; 95% CI: 75.0–94.8%) and longest radiographic progression-free survival time [26.6 months (95% CI: 20.9–not evaluable)]. Although this trial provides results consistent with the approved use of abiraterone acetate with prednisone, 5 mg, twice daily for the treatment of metastatic castration-resistant prostate cancer (mCRPC).
Generally, in clinical studies, 1 mg of dexamethasone is administered orally every day. Recently, Mehra et al. (70) evaluated the effects of low-dose steroids on neutrophil-to-lymphocyte ratio (NLR) in patients suffering from CRPC, and found treatment-naive CRPC patients with a high baseline or higher NLR values during treatment did not appear to benefit from low-dose corticosteroids. Zhu et al. (75) injected 10 mg of dexamethasone intravenously in 100 mL normal saline on the 1st day of the 3-week paclitaxel liposome protocol, and their PSA-based evaluation revealed that the therapy was effective in 14 cases (35%), and the median survival time was 17 months.
Over the last 5 years, prednisone was the most used steroid hormone in the endocrine therapy of CRPC. Recent studies have reported combinations with abiraterone acetate, docetaxel, cabazitaxel, Ra-223, orteronel, dutasteride, and apalutamide (77-84,92,93,95,96). Yu et al. (88) evaluated the effectiveness of intravenous apatorsen with oral prednisone (5 mg, twice daily) or prednisone alone, and observed a PSA decrease ≥50% in 47% of patients in the apatorsen with prednisone group compared to a decrease of 24% in the prednisone only group. In these reports, the use of steroid hormones was adjusted according to patients’ adverse reactions. However, few studies have compared dexamethasone or prednisone with a placebo. Thus, it is difficult to compare the efficacy of different steroid hormones.
Conclusions
Various GR isoforms may play an important part in the development of CRPC; however, the mechanism of CRPC remains complex and unclear. Most clinical trials in the last 5 years have focused on the use of prednisone. Only 1 clinical trial included a comparison of dexamethasone and hydrocortisone in its sequential treatment design. In short, GRs, including their isoforms, are involved in the progression of prostate cancer and may have implications on advanced treatment options. Further research is necessary to assess and attempt to understand and create hypotheses with GRs and the progression of prostate cancer more fully.
Acknowledgments
The authors appreciate the academic support from AME Prostate Cancer Collaborative Group.
Funding: None.
Footnote
Reporting Checklist: The authors have completed the Narrative Review reporting checklist. Available at https://tau.amegroups.com/article/view/10.21037/tau-22-501/rc
Conflicts of Interest: All authors have completed the ICMJE uniform disclosure form (available at https://tau.amegroups.com/article/view/10.21037/tau-22-501/coif). The authors have no conflicts of interest to declare.
Ethical Statement: The authors are accountable for all aspects of the work in ensuring that questions related to the accuracy or integrity of any part of the work are appropriately investigated and resolved.
Open Access Statement: This is an Open Access article distributed in accordance with the Creative Commons Attribution-NonCommercial-NoDerivs 4.0 International License (CC BY-NC-ND 4.0), which permits the non-commercial replication and distribution of the article with the strict proviso that no changes or edits are made and the original work is properly cited (including links to both the formal publication through the relevant DOI and the license). See: https://creativecommons.org/licenses/by-nc-nd/4.0/.
References
- Sung H, Ferlay J, Siegel RL, et al. Global Cancer Statistics 2020: GLOBOCAN Estimates of Incidence and Mortality Worldwide for 36 Cancers in 185 Countries. CA Cancer J Clin 2021;71:209-49. [Crossref] [PubMed]
- Huang Y, Jiang X, Liang X, et al. Molecular and cellular mechanisms of castration resistant prostate cancer. Oncol Lett 2018;15:6063-76. [Crossref] [PubMed]
- Valenca LB, Sweeney CJ, Pomerantz MM. Sequencing current therapies in the treatment of metastatic prostate cancer. Cancer Treat Rev 2015;41:332-40. [Crossref] [PubMed]
- Bishr M, Lattouf JB, Gannon PO, et al. Updates on therapeutic targets and agents in castration-resistant prostate cancer. Minerva Urol Nefrol 2011;63:131-43. [PubMed]
- Chandrasekar T, Yang JC, Gao AC, et al. Mechanisms of resistance in castration-resistant prostate cancer (CRPC). Transl Androl Urol 2015;4:365-80. [PubMed]
- Li Y, Yang R, Henzler CM, et al. Diverse AR Gene Rearrangements Mediate Resistance to Androgen Receptor Inhibitors in Metastatic Prostate Cancer. Clin Cancer Res 2020;26:1965-76. [Crossref] [PubMed]
- Ma T, Ungerleider N, Zhang DY, et al. Data of relative mRNA and protein abundances of androgen receptor splice variants in castration-resistant prostate cancer. Data Brief 2021;34:106774. [Crossref] [PubMed]
- Ma T, Bai S, Qi Y, et al. Increased transcription and high translation efficiency lead to accumulation of androgen receptor splice variant after androgen deprivation therapy. Cancer Lett 2021;504:37-48. [Crossref] [PubMed]
- Qu Y, Dai B, Ye D, et al. Constitutively active AR-V7 plays an essential role in the development and progression of castration-resistant prostate cancer. Sci Rep 2015;5:7654. [Crossref] [PubMed]
- Shorning BY, Dass MS, Smalley MJ, et al. The PI3K-AKT-mTOR Pathway and Prostate Cancer: At the Crossroads of AR, MAPK, and WNT Signaling. Int J Mol Sci 2020;21:4507. [Crossref] [PubMed]
- Tang D, He J, Dai Y, et al. Targeting KDM1B-dependent miR-215-AR-AGR2-axis promotes sensitivity to enzalutamide-resistant prostate cancer. Cancer Gene Ther 2022;29:543-57. [Crossref] [PubMed]
- Snipaitiene K, Bakavicius A, Lazutka JR, et al. Urinary microRNAs can predict response to abiraterone acetate in castration resistant prostate cancer: A pilot study. Prostate 2022;82:475-82. [Crossref] [PubMed]
- Lawrence MG, Porter LH, Choo N, et al. CX-5461 Sensitizes DNA Damage Repair-proficient Castrate-resistant Prostate Cancer to PARP Inhibition. Mol Cancer Ther 2021;20:2140-50. [Crossref] [PubMed]
- Hongo H, Kosaka T, Suzuki Y, et al. Topoisomerase II alpha inhibition can overcome taxane-resistant prostate cancer through DNA repair pathways. Sci Rep 2021;11:22284. [Crossref] [PubMed]
- Claessens F, Joniau S, Helsen C. Comparing the rules of engagement of androgen and glucocorticoid receptors. Cell Mol Life Sci 2017;74:2217-28. [Crossref] [PubMed]
- Narayanan S, Srinivas S, Feldman D. Androgen-glucocorticoid interactions in the era of novel prostate cancer therapy. Nat Rev Urol 2016;13:47-60. [Crossref] [PubMed]
- Wadosky KM, Koochekpour S. Molecular mechanisms underlying resistance to androgen deprivation therapy in prostate cancer. Oncotarget 2016;7:64447-70. [Crossref] [PubMed]
- Montgomery B, Cheng HH, Drechsler J, et al. Glucocorticoids and prostate cancer treatment: friend or foe? Asian J Androl 2014;16:354-8. [Crossref] [PubMed]
- Zhang C, Wenger T, Mattern J, et al. Clinical and mechanistic aspects of glucocorticoid-induced chemotherapy resistance in the majority of solid tumors. Cancer Biol Ther 2007;6:278-87. [Crossref] [PubMed]
- Yemelyanov A, Czwornog J, Chebotaev D, et al. Tumor suppressor activity of glucocorticoid receptor in the prostate. Oncogene 2007;26:1885-96. [Crossref] [PubMed]
- Rutz HP. Effects of corticosteroid use on treatment of solid tumours. Lancet 2002;360:1969-70. [Crossref] [PubMed]
- Isikbay M, Otto K, Kregel S, et al. Glucocorticoid receptor activity contributes to resistance to androgen-targeted therapy in prostate cancer. Horm Cancer 2014;5:72-89. [Crossref] [PubMed]
- Arora VK, Schenkein E, Murali R, et al. Glucocorticoid receptor confers resistance to antiandrogens by bypassing androgen receptor blockade. Cell 2013;155:1309-22. [Crossref] [PubMed]
- Hu J, Chen Q. The role of glucocorticoid receptor in prostate cancer progression: from bench to bedside. Int Urol Nephrol 2017;49:369-80. [Crossref] [PubMed]
- Hollenberg SM, Weinberger C, Ong ES, et al. Primary structure and expression of a functional human glucocorticoid receptor cDNA. Nature 1985;318:635-41. [Crossref] [PubMed]
- Lewis-Tuffin LJ, Cidlowski JA. The physiology of human glucocorticoid receptor beta (hGRbeta) and glucocorticoid resistance. Ann N Y Acad Sci 2006;1069:1-9. [Crossref] [PubMed]
- Oakley RH, Cidlowski JA. The biology of the glucocorticoid receptor: new signaling mechanisms in health and disease. J Allergy Clin Immunol 2013;132:1033-44. [Crossref] [PubMed]
- Kino T, Su YA, Chrousos GP. Human glucocorticoid receptor isoform beta: recent understanding of its potential implications in physiology and pathophysiology. Cell Mol Life Sci 2009;66:3435-48. [Crossref] [PubMed]
- Kino T, Chrousos GP. Glucocorticoid and mineralocorticoid receptors and associated diseases. Essays Biochem 2004;40:137-55. [Crossref] [PubMed]
- Wang K, Wang X, Fu X, et al. Lung cancer metastasis-related protein 1 promotes the transferring from advanced metastatic prostate cancer to castration-resistant prostate cancer by activating the glucocorticoid receptor α signal pathway. Bioengineered 2022;13:5373-85. [Crossref] [PubMed]
- Xu M, Martinez G, Kipp Z, et al. Glucocorticoid Receptor Beta as a Contributor to Prostate Cancer Growth and Migration. FASEB J 2022;36. Available online:
10.1096/fasebj.2022.36.S1.R5303 10.1096/fasebj.2022.36.S1.R5303 - Ramos-Ramírez P, Tliba O. Glucocorticoid Receptor β (GRβ): Beyond Its Dominant-Negative Function. Int J Mol Sci 2021;22:3649. [Crossref] [PubMed]
- Lewis-Tuffin LJ, Jewell CM, Bienstock RJ, et al. Human glucocorticoid receptor beta binds RU-486 and is transcriptionally active. Mol Cell Biol 2007;27:2266-82. [Crossref] [PubMed]
- Ligr M, Li Y, Logan SK, et al. Mifepristone inhibits GRβ coupled prostate cancer cell proliferation. J Urol 2012;188:981-8. [Crossref] [PubMed]
- Jacob A, Raj R, Allison DB, et al. Androgen Receptor Signaling in Prostate Cancer and Therapeutic Strategies. Cancers (Basel) 2021;13:5417. [Crossref] [PubMed]
- Puhr M, Hoefer J, Eigentler A, et al. The Glucocorticoid Receptor Is a Key Player for Prostate Cancer Cell Survival and a Target for Improved Antiandrogen Therapy. Clin Cancer Res 2018;24:927-38. [Crossref] [PubMed]
- Sahu B, Laakso M, Pihlajamaa P, et al. FoxA1 specifies unique androgen and glucocorticoid receptor binding events in prostate cancer cells. Cancer Res 2013;73:1570-80. [Crossref] [PubMed]
- Wu M, Xie Y, Cui X, et al. Rational drug design for androgen receptor and glucocorticoids receptor dual antagonist. Eur J Med Chem 2019;166:232-42. [Crossref] [PubMed]
- Serritella AV, Shevrin D, Heath EI, et al. Phase I/II Trial of Enzalutamide and Mifepristone, a Glucocorticoid Receptor Antagonist, for Metastatic Castration-Resistant Prostate Cancer. Clin Cancer Res 2022;28:1549-59. [Crossref] [PubMed]
- Motavalli R, Majidi T, Pourlak T, et al. The clinical significance of the glucocorticoid receptors: Genetics and epigenetics. J Steroid Biochem Mol Biol 2021;213:105952. [Crossref] [PubMed]
- Boldizsar F, Talaber G, Szabo M, et al. Emerging pathways of non-genomic glucocorticoid (GC) signalling in T cells. Immunobiology 2010;215:521-6. [Crossref] [PubMed]
- Buttgereit F, Scheffold A. Rapid glucocorticoid effects on immune cells. Steroids 2002;67:529-34. [Crossref] [PubMed]
- Joseph JD, Lu N, Qian J, et al. A clinically relevant androgen receptor mutation confers resistance to second-generation antiandrogens enzalutamide and ARN-509. Cancer Discov 2013;3:1020-9. [Crossref] [PubMed]
- Miller WL, Auchus RJ. The molecular biology, biochemistry, and physiology of human steroidogenesis and its disorders. Endocr Rev 2011;32:81-151. [Crossref] [PubMed]
- Cain DW, Cidlowski JA. Immune regulation by glucocorticoids. Nat Rev Immunol 2017;17:233-47. [Crossref] [PubMed]
- Strehl C, van der Goes MC, Bijlsma JW, et al. Glucocorticoid-targeted therapies for the treatment of rheumatoid arthritis. Expert Opin Investig Drugs 2017;26:187-95. [Crossref] [PubMed]
- Oray M, Abu Samra K, Ebrahimiadib N, et al. Long-term side effects of glucocorticoids. Expert Opin Drug Saf 2016;15:457-65. [Crossref] [PubMed]
- Noetzlin S, Breville G, Seebach JD, et al. Short-term glucocorticoid-related side effects and adverse reactions: a narrative review and practical approach. Swiss Med Wkly 2022;152:w30088. [PubMed]
- Elena C, Chiara M, Angelica B, et al. Hyperglycemia and Diabetes Induced by Glucocorticoids in Nondiabetic and Diabetic Patients: Revision of Literature and Personal Considerations. Curr Pharm Biotechnol 2018;19:1210-20. [Crossref] [PubMed]
- Perez A, Jansen-Chaparro S, Saigi I, et al. Glucocorticoid-induced hyperglycemia. J Diabetes 2014;6:9-20. [Crossref] [PubMed]
- Cui A, Fan H, Zhang Y, et al. Dexamethasone-induced Krüppel-like factor 9 expression promotes hepatic gluconeogenesis and hyperglycemia. J Clin Invest 2019;129:2266-78. [Crossref] [PubMed]
- Broersen LH, Pereira AM, Jørgensen JO, et al. Adrenal Insufficiency in Corticosteroids Use: Systematic Review and Meta-Analysis. J Clin Endocrinol Metab 2015;100:2171-80. [Crossref] [PubMed]
- Van Staa TP, Laan RF, Barton IP, et al. Bone density threshold and other predictors of vertebral fracture in patients receiving oral glucocorticoid therapy. Arthritis Rheum 2003;48:3224-9. [Crossref] [PubMed]
- Da Silva JA, Jacobs JW, Kirwan JR, et al. Safety of low dose glucocorticoid treatment in rheumatoid arthritis: published evidence and prospective trial data. Ann Rheum Dis 2006;65:285-93. [Crossref] [PubMed]
- Lambrou GI, Adamaki M, Hatziagapiou K, et al. Gene Expression and Resistance to Glucocorticoid-Induced Apoptosis in Acute Lymphoblastic Leukemia: A Brief Review and Update. Curr Drug Res Rev 2020;12:131-49. [Crossref] [PubMed]
- Hulkko SM, Wakui H, Zilliacus J. The pro-apoptotic protein death-associated protein 3 (DAP3) interacts with the glucocorticoid receptor and affects the receptor function. Biochem J 2000;349:885-93. [Crossref] [PubMed]
- Hulkko SM, Zilliacus J. Functional interaction between the pro-apoptotic DAP3 and the glucocorticoid receptor. Biochem Biophys Res Commun 2002;295:749-55. [Crossref] [PubMed]
- Wang X, Shi J, Gong D. Mometasone furoate inhibits growth of acute leukemia cells in childhood by regulating PI3K signaling pathway. Hematology 2018;23:478-85. [Crossref] [PubMed]
- Rhen T, Cidlowski JA. Antiinflammatory action of glucocorticoids--new mechanisms for old drugs. N Engl J Med 2005;353:1711-23. [Crossref] [PubMed]
- Coutinho AE, Chapman KE. The anti-inflammatory and immunosuppressive effects of glucocorticoids, recent developments and mechanistic insights. Mol Cell Endocrinol 2011;335:2-13. [Crossref] [PubMed]
- Tannock I, Gospodarowicz M, Meakin W, et al. Treatment of metastatic prostatic cancer with low-dose prednisone: evaluation of pain and quality of life as pragmatic indices of response. J Clin Oncol 1989;7:590-7. [Crossref] [PubMed]
- Montgomery B, Kheoh T, Molina A, et al. Impact of baseline corticosteroids on survival and steroid androgens in metastatic castration-resistant prostate cancer: exploratory analysis from COU-AA-301. Eur Urol 2015;67:866-73. [Crossref] [PubMed]
- Woods-Burnham L, Cajigas-Du Ross CK, Love A, et al. Glucocorticoids Induce Stress Oncoproteins Associated with Therapy-Resistance in African American and European American Prostate Cancer Cells. Sci Rep 2018;8:15063. [Crossref] [PubMed]
- Djeu JY, Wei S. Clusterin and chemoresistance. Adv Cancer Res 2009;105:77-92. [Crossref] [PubMed]
- Chun YJ. Knockdown of clusterin expression increases the in vitro sensitivity of human prostate cancer cells to paclitaxel. J Toxicol Environ Health A 2014;77:1443-50. [Crossref] [PubMed]
- Ríos-Colón L, Cajigas-Du Ross CK, Basu A, et al. Targeting the stress oncoprotein LEDGF/p75 to sensitize chemoresistant prostate cancer cells to taxanes. Oncotarget 2017;8:24915-31. [Crossref] [PubMed]
- Ortiz-Hernandez GL, Sanchez-Hernandez ES, Casiano CA. Twenty years of research on the DFS70/LEDGF autoantibody-autoantigen system: many lessons learned but still many questions. Auto Immun Highlights 2020;11:3. [Crossref] [PubMed]
- Ortiz-Hernandez GL, Sanchez-Hernandez ES, Ochoa PT, et al. The LEDGF/p75 Integrase Binding Domain Interactome Contributes to the Survival, Clonogenicity, and Tumorsphere Formation of Docetaxel-Resistant Prostate Cancer Cells. Cells 2021;10:2723. [Crossref] [PubMed]
- Foroughi Moghadam MJ, Taheri S, Peiravian F. A Systematic Review of Clinical Practice Guidelines for Castration-Resistant Prostate Cancer. Iran J Pharm Res 2018;17:17-37. [PubMed]
- Mehra N, Sharp A, Lorente D, et al. Neutrophil to Lymphocyte Ratio in Castration-Resistant Prostate Cancer Patients Treated With Daily Oral Corticosteroids. Clin Genitourin Cancer 2017;15:678-684.e1. [Crossref] [PubMed]
- Tanaka N, Nishimura K, Okajima E, et al. The efficacy and safety of docetaxel-based chemotherapy combined with dexamethasone 1 mg daily oral administration: JMTO Pca 10-01 phase II trial. Jpn J Clin Oncol 2017;47:247-51. [Crossref] [PubMed]
- Calvani N, Morelli F, Naglieri E, et al. Metronomic chemotherapy with cyclophosphamide plus low dose of corticosteroids in advanced castration-resistant prostate cancer across the era of taxanes and new hormonal drugs. Med Oncol 2019;36:80. [Crossref] [PubMed]
- Nakai Y, Tanaka N, Ichikawa K, et al. Appropriate Number of Docetaxel Cycles in Castration-Resistant Prostate Cancer Patients Considering Peripheral Neuropathy and Oncological Control. Chemotherapy 2020;65:119-24. [Crossref] [PubMed]
- Noguchi M, Arai G, Egawa S, et al. Mixed 20-peptide cancer vaccine in combination with docetaxel and dexamethasone for castration-resistant prostate cancer: a randomized phase II trial. Cancer Immunol Immunother 2020;69:847-57. [Crossref] [PubMed]
- Zhu SS, Zhang FY, Xue S, et al. Paclitaxel liposome for the treatment of castration-resistant prostate cancer. Zhonghua Nan Ke Xue 2020;26:788-92. [PubMed]
- Roviello G, Petrioli R, Bonetta A, et al. Corticosteroid switch in heavily pre-treated castration-resistant prostate cancer patients progressed on abiraterone acetate plus prednisone. Invest New Drugs 2018;36:1110-5. [Crossref] [PubMed]
- Fizazi K, Jones R, Oudard S, et al. Phase III, randomized, double-blind, multicenter trial comparing orteronel (TAK-700) plus prednisone with placebo plus prednisone in patients with metastatic castration-resistant prostate cancer that has progressed during or after docetaxel-based therapy: ELM-PC 5. J Clin Oncol 2015;33:723-31. [Crossref] [PubMed]
- Petrylak DP, Gandhi JG, Clark WR, et al. Phase 1/2 study of orteronel (TAK-700), an investigational 17,20-lyase inhibitor, with docetaxel-prednisone in metastatic castration-resistant prostate cancer. Invest New Drugs 2015;33:397-408. [Crossref] [PubMed]
- Shore ND. Open-label phase II study evaluating the efficacy of concurrent administration of radium Ra 223 dichloride (Ra-223) and abiraterone acetate (AA) in men with castration-resistant prostate cancer (CRPC) patients with symptomatic bone metastases. J Clin Oncol 2016;34:177. [Crossref]
- Eisenberger M, Hardy-Bessard AC, Kim CS, et al. Phase III Study Comparing a Reduced Dose of Cabazitaxel (20 mg/m 2) and the Currently Approved Dose (25 mg/m 2) in Postdocetaxel Patients With Metastatic Castration-Resistant Prostate Cancer-PROSELICA. J Clin Oncol 2017;35:3198-206. [Crossref] [PubMed]
- Friedlander TW, Graff JN, Zejnullahu K, et al. High-Dose Abiraterone Acetate in Men With Castration Resistant Prostate Cancer. Clin Genitourin Cancer 2017;15:733-741.e1. [Crossref] [PubMed]
- Massard C, Mateo J, Loriot Y, et al. Phase I/II trial of cabazitaxel plus abiraterone in patients with metastatic castration-resistant prostate cancer (mCRPC) progressing after docetaxel and abiraterone. Ann Oncol 2017;28:90-5. [Crossref] [PubMed]
- McKay RR, Werner L, Mostaghel EA, et al. A Phase II Trial of Abiraterone Combined with Dutasteride for Men with Metastatic Castration-Resistant Prostate Cancer. Clin Cancer Res 2017;23:935-45. [Crossref] [PubMed]
- van Dodewaard-de Jong JM, de Klerk JMH, Bloemendal HJ, et al. A randomised, phase II study of repeated rhenium-188-HEDP combined with docetaxel and prednisone versus docetaxel and prednisone alone in castration-resistant prostate cancer (CRPC) metastatic to bone; the Taxium II trial. Eur J Nucl Med Mol Imaging 2017;44:1319-27. [Crossref] [PubMed]
- Heller G, McCormack R, Kheoh T, et al. Circulating Tumor Cell Number as a Response Measure of Prolonged Survival for Metastatic Castration-Resistant Prostate Cancer: A Comparison With Prostate-Specific Antigen Across Five Randomized Phase III Clinical Trials. J Clin Oncol 2018;36:572-80. [Crossref] [PubMed]
- Mehra N, Dolling D, Sumanasuriya S, et al. Plasma Cell-free DNA Concentration and Outcomes from Taxane Therapy in Metastatic Castration-resistant Prostate Cancer from Two Phase III Trials (FIRSTANA and PROSELICA). Eur Urol 2018;74:283-91. [Crossref] [PubMed]
- Szmulewitz RZ, Peer CJ, Ibraheem A, et al. Prospective International Randomized Phase II Study of Low-Dose Abiraterone With Food Versus Standard Dose Abiraterone In Castration-Resistant Prostate Cancer. J Clin Oncol 2018;36:1389-95. [Crossref] [PubMed]
- Yu EY, Ellard SL, Hotte SJ, et al. A randomized phase 2 study of a HSP27 targeting antisense, apatorsen with prednisone versus prednisone alone, in patients with metastatic castration resistant prostate cancer. Invest New Drugs 2018;36:278-87. [Crossref] [PubMed]
- Hakenberg OW, Perez-Gracia JL, Castellano D, et al. Randomised phase II study of second-line olaratumab with mitoxantrone/prednisone versus mitoxantrone/prednisone alone in metastatic castration-resistant prostate cancer. Eur J Cancer 2019;107:186-95. [Crossref] [PubMed]
- Lorente D, Mejorada RL, De de Rueda GAO, et al. 864P - External validation of a prognostic score in first-line metastastic castration-resistant prostate cancer (mCRPC). Ann Oncol 2019;30:v337-8. [Crossref]
- Tagawa ST, Antonarakis ES, Gjyrezi A, et al. Expression of AR-V7 and ARv567es in Circulating Tumor Cells Correlates with Outcomes to Taxane Therapy in Men with Metastatic Prostate Cancer Treated in TAXYNERGY. Clin Cancer Res 2019;25:1880-8. [Crossref] [PubMed]
- Posadas EM, Chi KN, de Wit R, et al. Pharmacokinetics, Safety, and Antitumor Effect of Apalutamide with Abiraterone Acetate plus Prednisone in Metastatic Castration-Resistant Prostate Cancer: Phase Ib Study. Clin Cancer Res 2020;26:3517-24. [Crossref] [PubMed]
- Attard G, Merseburger AS, Arlt W, et al. Assessment of the Safety of Glucocorticoid Regimens in Combination With Abiraterone Acetate for Metastatic Castration-Resistant Prostate Cancer: A Randomized, Open-label Phase 2 Study. JAMA Oncol 2019;5:1159-67. [Crossref] [PubMed]
- Attard G, Murphy L, Clarke NW, et al. Abiraterone acetate and prednisolone with or without enzalutamide for high-risk non-metastatic prostate cancer: a meta-analysis of primary results from two randomised controlled phase 3 trials of the STAMPEDE platform protocol. Lancet 2022;399:447-60. [Crossref] [PubMed]
- George DJ, Halabi S, Heath EI, et al. A prospective trial of abiraterone acetate plus prednisone in Black and White men with metastatic castrate-resistant prostate cancer. Cancer 2021;127:2954-65. [Crossref] [PubMed]
- Heiss BL, Geynisman DM, Martinez E, et al. Comparison of out-of-pocket costs and adherence between the two arms of the prospective, randomized abiraterone food effect trial. Support Care Cancer 2022;30:2803-10. [Crossref] [PubMed]
(English Language Editor: L. Huleatt)