Morphofunctional characteristics of proliferation and apoptosis of germinal epithelium: pathogenesis of temporary azoospermia after local electron irradiation
Highlight box
Key findings
• The basis of focal hypospermatogenesis is the electron irradiation-induced proliferative-apoptotic imbalance. This leads to temporary azoospermia.
What is known and what is new?
• Violations of spermatogenesis in other types of ionizing radiation were studied.
• We studied the effect of electron irradiation on spermatogenesis and clarified the mechanisms of development of temporary radiation-induced azoospermia. This is due to a violation of the proliferative-apoptotic balance of germ cells and their subsequent death.
What is the implication, and what should change now?
• The results of this study can be used to select the most effective/safe doses of electron irradiation.
Introduction
The polyetiology and complexity of the mechanisms of pathological spermatogenesis, the close functional dependence of the testes on the action of various factors create considerable difficulties in diagnosis, the development of adequate methods of treatment and prevention (1,2).
With the introduction of new diagnostic and therapeutic methods in radiobiology and radiation therapy, the question arises of their safety for humans, including for their reproductive function. The data of the specialized literature on the effects of ionizing radiation on the testis are ambiguous. On the one hand, radiation therapy has been proven to entail the risk of infertility, however, on the other hand, after radiation therapy, type A spermatogonia may remain in the testicles, which in some cases restore spermatogenesis (3).
During studying the pathogenetic mechanisms of male infertility, various models are used on laboratory animals, followed by extrapolation of the results to humans. When modeling, drugs from the group of cytostatics or ionizing radiation (β-, γ-, X-rays, etc.) are used as gonadotoxic factors, leading to disruption of DNA replication and RNA transcription, which suppresses the cell cycle of male gametes (3-5). The indicator of these changes, first of all, is the assessment of proliferation and apoptosis of germ cells, which are regulated by Ki-67 proteins and caspases (6,7). It is known that the germinal epithelium is highly sensitive to radioactive radiation, which is manifested by a decrease in mitotic division and the death of germ cells, especially spermatogonia (8). Apoptosis is activated via extrinsic and intrinsic pathways, and caspase-3 is responsible for the terminal phase. Other cell cycle regulators are proapoptotic (p53) and antiapoptotic (Bcl-2) factors (6).
Thus, at present, the imbalance between proliferation and apoptosis caused by various types of irradiation and, in particular, by electrons, is caused by damage to various stages of the life cycle of germ cells and remains relevant.
The purpose of the study was to evaluate the cell cycle of germ cells after electron irradiation with a dose of 2 Gy. Objectives of this study was to assess the expression levels of the universal proliferation factor Ki-67, apoptosis termination factor (caspase 3), key factors of anti-apoptosis (Bcl-2) and pro-apoptosis (p53), as well as DNA fragmentation [Terminal deoxynucleotidyl transferase (TdT) dUTP Nick-End Labeling (TUNEL)]. This article is presented in accordance with the ARRIVE reporting checklist (available at https://tau.amegroups.com/article/view/10.21037/tau-22-598/rc).
Methods
An experimental morphological study was carried out on the basis of the Medical Radiological Scientific Center named after A.F. Tsyb and Sechenov University.
Animals for in vivo research
Wistar rats (n=60) were divided into groups (conventional names): (I) control (n=30), which were injected with saline; (II) single local irradiation of the testes with electrons at a dose of 2 Gy (2IR; dose rate 1 Gy/min, energy 10 MeV and frequency 9 Hz, field size—Ø 100 mm, linear accelerator “NOVAC-11”) (n=30). Animals were gradually withdrawn from the experiment over 11 weeks: 5 animals one week after irradiation, and then 5 animals once every 2 weeks.
All manipulations were carried out in accordance with the International Recommendations for Biomedical Research Using Animals [Eurasian Economic Commission (EEC), Strasbourg, 1985], the European Convention for the Protection of Vertebrate Animals used for Experimental or other Scientific Purposes (EEC, Strasbourg, 1986), and Guidelines for Medical-biological research on the care and use of laboratory animals [Institute for Laboratory Animal Research (ILAR), Division on Earth and Life Studies (DELS)]. Experiments were performed under a project license (No. 043 dated August 11, 2020) granted by institutional ethics committee of Sechenov University, in compliance with national and institutional guidelines for the care and use of animals.
After extraction, testes were cut parallel to the sagittal plane every 2 mm, fixed in Bouin’s solution, after insertion (apparatus for histological tissue guiding, Leica Biosystems, Germany), they were embedded in paraffin blocks, from which serial sections (3 µm thick) were prepared, dewaxed, dehydrated and stained hematoxylin and eosin for histological examination.
The following parameters were calculated in damaged seminiferous tubules: the height of the germinal epithelium, the number of germ cells. The number of Leydig cells was determined by computer morphometry in 10 fields of view with a total area of 1.6 mm2.
Testes after fixation in Bouin’s solution for immunohistochemical (IHC) studies were prepared according to the standard protocol. Antigen unmasking was carried out in citrate buffer, pH 6.0. Monoclonal antibodies to Ki-67 (Clone MM1; 1:200), Caspase-3 (Clone ab2302, 1:300), Bcl-2 (Clone bcl-2/100/D5; 1:50) and p53 were used as primary antibodies. (Clone DO-7; 1:200), and the secondary ones were the universal two-component detection system HiDef DetectionTM HRP Polymer system (Cell Marque, USA), mouse/rabbit anti-immunoglobulin G (IGG), horseradish peroxidase (HRP), and 3,3’-diaminobenzidine (DAB) substrate. Cell nuclei were counterstained with Mayer’s hematoxylin.
The number of immunopositive cells was counted in 10 randomly selected fields of view at ×400 magnification (in percent).
Microscopic analysis was performed using a video microscopy system (microscope Leica DM2000, Germany; camera Leica ICC50 HD).
TUNEL method was used to study DNA fragmentation in all types of germ cells, which were stained with TdT solution (Thermo Fisher, USA) and incubated for 60 minutes. The nuclei were counterstained with 4’,6-diamidino-2-phenylindole (DAPI) (blue spectrum) (Thermo Fisher), and the luminescence intensity was controlled in a fluorescent microscope with a set of fluorescein isothiocyanate (FITC) filters (green spectrum).
Statistical analysis
The obtained data were processed using the SPSS 12 for Windows statistical software package (IBM Analytics, USA). The arithmetic mean values with its limiting deviations and the standard error were calculated. Comparison between groups was performed using one-way analysis of variance (ANOVA) with P<0.01 significance.
Results
This section describes the features of proliferative, apoptotic and reparative processes in the testes from the point of view of the interaction between the germinal and endocrine components in experimental hypospermatogenesis caused by local electron irradiation in comparison with the control.
The results below (height of the germinal epithelium, the number of germ cells, Sertoli cells and Leydig cells) were obtained by morphometric analysis of transverse serial sections of the testes. To assess the degree of damage to the seminiferous tubules, only destructively altered seminiferous tubules were taken into account.
Histologic assessment
In control group was observed physiological spermatogenesis. In testes of irradiated animals was discovered morphological picture of azoospermia characterized by focal destruction and wrinkling of the seminiferous tubules, initial signs of germinal aplasia with segmental vacuolization of the germinal epithelium. The share of damaged seminiferous tubules with disorganization of the germinal epithelium accounted for up to 1/8 of the area of the testis. At the same time, Sertoli cells were well identified, especially mature forms, by the structure of the nucleus and the centrally located nucleolus. In the intertubular space, there were signs of interstitial edema and stasis in the lumen of blood vessels; dilated lymphatic vessels. At the 3rd week degenerative spermatids and spermatocytes were found, combined into giant single-nuclear and multinuclear cells (Ø 0.4–0.6 µm)—large structures with multiple pycnotic nuclei and intensely stained oxyphilic cytoplasm. At the 7th week the share of destructive seminiferous tubules accounted for up to 1/4 of each of the studied sections of the testis, and by the end of the experiment, it amounted to 1/3 of the part. The number of multinuclear giant cells gradually decreased (Figure 1).
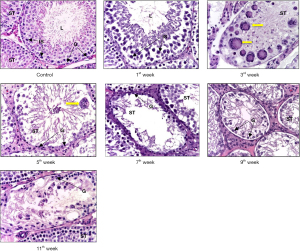
It should be noted that in the group of irradiated animals, at the 11th week a restoration of the number of germ cells was revealed, mainly due to spermatogonia and spermatocytes.
Morphometric analysis
The height of the germinal epithelium in the damaged seminiferous tubules gradually decreased throughout the experiment. By the end of the experiment, this figure was 1.3 times less compared to the control (P<0.01) (Table 1).
Table 1
Groups | Height of germinal epithelium, µm | Spermatogonia | Spermatocytes | Spermatids |
---|---|---|---|---|
Control | 344.1±13.2 | 28.2±4.2 | 128.3±4.5 | 274.9±5.8 |
2IR 1st week | 273.7±12.9 | 22.8±1.1 | 83.4±4.0 | 134.7±6.6 |
2IR 3rd week | 269.1±10.5 | 10.2±0.4 | 37.8±1.7 | 69.8±3.2 |
2IR 5th week | 265.6±11.4 | 4.3±0.2 | 21.1±0.7 | 34.1±1.6 |
2IR 7th week | 261.8±9.2 | 2.1±0.1 | 15.3±0.6 | 28.2±1.2 |
2IR 9th week | 254.3±10.7 | 1.8±0.08 | 7.9±0.3 | 16.9±0.6 |
2IR 11th week | 247.7±9.7 | 12.2±0.5 | 6.1±0.2 | 7.1±0.2 |
2IR, ionizing radiation at a dose 2 Gy.
After irradiation a decrease in the number of germ cells was observed. Throughout the experiment in the testis samples were revealed a gradual decrease in the number of germ cells, signs of degenerative changes and lysis of spermatids and spermatozoa, as well as the appearance, in some places, of pyknotic nuclei in primary spermatocytes (Table 1). Unchanged number of Sertoli cells was observed. Hyperplasia of the Leydig cells without changing their structure was observed (Table 2).
Table 2
Groups | Sertoli cells, in destructive tubule | Leydig cells, in 1 mm2 |
---|---|---|
Control | 19.7±0.6 | 145±7.0 |
2IR 1st week | 18.5±0.02 | 152±7.5 |
2IR 3rd week | 17.6±0.02 | 149±7.1 |
2IR 5th week | 17.2±0.01 | 153±7.5 |
2IR 7th week | 16.6±0.01 | 167±7.9 |
2IR 9th week | 16.1±0.07 | 201±9.7 |
2IR 11th week | 15.4±0.07 | 242±12.1 |
2IR, ionizing radiation at a dose 2 Gy.
IHC assessment
A week after irradiation, the number of Ki-67-positive germ cells in the germinal epithelium was 1.4 times lower than in group I. Then, a constant decrease in the number of Ki-67-positive germ cells was observed: at the 3rd week—2.5 times, at the 7th week—3.6 times and at the 11th week—4.6 times compared with the control. On the contrary, Leydig cell hyperplasia was accompanied by an increase in the number of Ki-67-positive Leydig cells throughout the experiment and by the end of it was 1.7 times more than in the control (Table 3, Figure 2).
Table 3
Group | Ki-67, % | Bcl-2, % | p53, % | Caspase 3, % |
---|---|---|---|---|
Control | 76.0±3.7 | 53.4±2.6 | 54.3±2.7 | 5.0±0.2 |
2IR 1st week | 53.7±2.6 | 29.3±1.3 | 57.1±2.8 | 12.3±0.5 |
2IR 3rd week | 34.2±1.6 | 15.2±0.7 | 65.4±3.1 | 12.5±0.5 |
2IR 5th week | 29.7±1.4 | 14.5±0.7 | 66.7±3.2 | 12.9±0.6 |
2IR 7th week | 24.9±1.2 | 13.7±0.6 | 68.2±3.3 | 13.2±0.5 |
2IR 9th week | 21.4±1.0 | 12.5±0.6 | 69.9±3.4 | 13.8±0.6 |
2IR 11th week | 19.5±0.7 | 11.6±0.5 | 71.5±3.5 | 14.5±0.6 |
2IR, ionizing radiation at a dose 2 Gy.
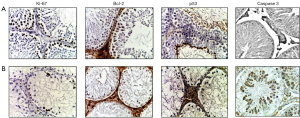
The number of Bcl-2-stained germ cells (single spermatogonia, spermatocytes and spermatids) on the first week was 1.8 times lower than the control values and continued to decrease: at the 3rd week—by 3.7 times, at the 7th week—by 4.3 times and by the end of the experiment—5.9 times less than in the control group. At the same time, the number of Leydig cells after irradiation was absent in the first week, and then slightly increased: at the 3rd week—by 1.4 times, at the 7th week—by 4.2 times, and at the 11th week—by 7.2 times compared with the first group (Table 3, Figure 2).
The number of p53-stained germ cells in the first week after electron irradiation showed some tendency to increase (mainly due to spermatogonia) compared with the 1st group, which was dominated by p53-stained spermatids and spermatozoa. Then, their number slightly increased: at the 3rd week—1.2 times, at the 7th week—1.29 times, and at the 11th week—1.38 times compared with the control. At the 1st week, single stained Leydig cells were found and then at the 3rd week the p53-positive Leydig cells disappeared. At the 7th week they reappeared and were 2.5 times larger than in the control group, and disappeared again at the 11th week (Table 3, Figure 2).
A week after irradiation, the number of Cas-3-positive germ cells was 5.46 times higher than in group I. Then, a constant increase in the number of Cas-3-positive germ cells was observed: at the 3rd week—5.56 times, at the 7th week—5.92 times and at the 11th week—6.14 times compared with the control. On the contrary, the number of Cas-3-positive Leydig cells decreased slightly throughout the experiment (Table 3, Figure 2).
TUNEL
In group I, during the experiment, TUNEL-positive cells accounted for 16.0%. In group II, a week after electron irradiation, their number (mainly spermatogonia) increased 1.4 times compared with the control. At the 3rd week after irradiation the number of TUNEL-positive germ cells (mainly spermatogonia) in the II group increased by 1.6 times, at the 7th week—by 3.4 times (mainly among primary spermatocytes), and at the end of the experiment—by 4.6 times compared to control. It is important to note that among the small number of surviving germ cells, 75% were TUNEL-positive by the end of the experiment, that is, almost all. A gradual increase in the number of TUNEL-positive Leydig cells was also observed (Figure 3).
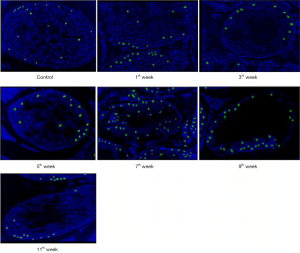
Discussion
This study is devoted to disorders in the proliferation and apoptosis of germ cells that have arisen after exposure to ionizing radiation on spermatogenesis.
For the first time, electron irradiation was chosen as an inducer of hypospermatogenesis. Moreover, local electron irradiation in our study was the most targeted. The unique combination (single electron irradiation, dose 2 Gy, rat model) is an important feature of our study in the comparative morphological evaluation of spermatogenesis over time. The selected dose of 2 Gy is the base dose in clinical practice for single and fractional irradiation. This allows us to extrapolate our data to humans.
After local electron irradiation with a dose of 2 Gy, a pronounced decrease in the number of Ki-67- and Bcl-2-positive germ cells was observed against the background of an increase in the number of caspase-3- and p53-positive cells.
The pathogenesis of exposure to electron irradiation in the testes is based on the pathomorphological changes found during the study and partially supplemented by data from specialized literature and includes two main development paths: direct and indirect (Figure 4) (9).
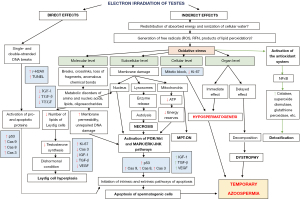
Electrons penetrating the nucleus of germ cells (direct action), primarily actively dividing (spermatogonia), cause single- and double-stranded DNA breaks: reversible (repairable) and irreversible (non-repairable), activating pro- and apoptotic proteins predominantly of the internal pathway of apoptosis and is one of the main factors, which subsequently leads to the death of germ cells and, as a result, to temporary azoospermia (9).
Taking into account the high levels of DNA fragmentation in the germ cells according to the TUNEL results and the histological picture of hypospermatogenesis after electron irradiation with a dose of 2 Gy against the background of relatively low expression of caspase-3, it is possible to speak with a high degree of probability about the activation of not only the caspase cascade characteristic of classical apoptosis, but also about other types of non-apoptotic cell death, for example, necrosis dependent on mitochondrial permeability (MPT-driven necrosis, mitochondrial permeability transition-driven necrosis) or paraptosis (10).
Another mechanism leading to a decrease in the pool of germ cells is explained by the indirect effects of electron irradiation, as a result of which the absorbed energy is redistributed in cells between different molecules and substances, as well as the ionization of intracellular water molecules. This entails a pronounced generation of a large amount of free radicals (reactive oxygen species, reactive forms of nitrogen, products of lipid peroxidation and water ionization), the accumulation of which in the cell is commonly called oxidative stress, in response to which physiological antioxidant mechanisms are triggered. The cell synthesizes a large number of detoxifying enzymes (catalases, glutathione peroxidases, glutathione-S-transferases, superoxide dismutases), accumulates high concentrations of low molecular weight endogenous antioxidants, such as ubiquinone, glutathione, ascorbate, lipoic acid and vitamin E (9). Against this background, an even greater amount of free radicals is produced. as a result of the decay of previously accumulated reactive substances. All of the above leads to the dominance of the mechanisms of oxidative stress over the antioxidant system, a shift in the redox balance towards oxidation and insufficiency of the detoxifying system of the cell, which ultimately leads to damage at all levels.
At the molecular level: a violation of the metabolism of amino acids, nucleic acids, oligosaccharides, polyunsaturated fatty acids and other lipids (their destruction—breaks and crosslinks, the formation of abnormal chemical bonds, loss or exchange of molecular sections). The destruction of lipid molecules, which normally form the cell membrane, is critical, contributing to an increase in its permeability and disruption of homeostasis, which in turn leads to the activation of the Phosphatidylinositol-3-Kinase and Protein Kinase B (PI3K/Akt) and mitogen-activated protein kinase/extracellular signal-regulated kinases/c-Jun N-terminal kinase (MAPK/ERK/JNK) signaling pathways, triggering the caspase cascade, and apoptosis of germ cells. This is accompanied by damage to lipids deposited in large quantities in the vesicles of Leydig cells as a source of cholesterol for the synthesis of sex hormones, and, as a result, a decrease in testosterone synthesis. This leads to the development of a dishormonal state, in response to which Leydig cells respond with active proliferation (hyperplasia) to increase the concentration of testosterone in the blood plasma.
At the subcellular level, biological membranes are destroyed. Destruction of the nuclear membrane, accompanied by damage to the genetic material, activates the previously described signaling pathways and triggers apoptosis. The release of lysosome enzymes into the cytoplasm as a result of the destruction of their membrane entails autolysis and necrosis. Damage to mitochondria leads to a decrease in ATP synthesis due to the destruction of the electron transport chain and the development of a hypoenergetic state with a predominance of catabolism and a violation of the trophic function of the cell, which is also the reason for the activation of apoptotic cascades. It should be noted that the destruction of the outer and inner membranes of mitochondria probably triggers cell death by the type of necrosis dependent on mitochondrial permeability (10).
At the cellular level, electron irradiation leads to inhibition of the life cycle of germ cells, which is manifested by a decrease in their number—hypospermatogenesis. This is accompanied by a decrease in height and vacuolization of the germinal epithelium, a decrease in the diameter and cross-sectional area of the seminiferous tubule, their destruction, as well as an increase in the area and edema of the interstitial tissue (11,12).
According to some authors, after exposure to γ-rays of Co60 at a dose of 2 Gy on the testes of rats (irradiation of the pelvic segment), already on the 3rd day, a decrease in the diameter of the seminiferous tubules by 2.0 times and the height of the spermatogenic epithelium by 1.6 was found, while in our study on first week after electron irradiation, these parameters were reduced only by 1.1 and 1.25 times, respectively (13). In another study, it was shown that after irradiation of the pelvic segment in rats with X-rays at a dose of 2 Gy, the following signs were found after 7 days: the appearance of atrophic convoluted seminiferous tubules, disorganization and vacuolization of the germinal epithelium, pycnosis of nuclei of spermatogonia and spermatocytes, edema and stasis of blood vessels of the interstitial tissue (14), while in our study, after electron irradiation at a dose of 2 Gy, these pathomorphological changes were observed only on the 3rd week of the experiment.
The duration of the experiment (11 weeks) makes it possible to evaluate the dynamics of the development of post-radiation effects in the testes during 2–3 cycles of rat spermatogenesis. Already by the end of the experiment, there were signs of initial restoration of spermatogenesis (a slight increase in the number of spermatogonia). Based on some studies, X- and gamma-ray damage persisted for more than 8 months without signs of recovery of the germinal epithelium (15). Thus, signs of restoration of spermatogenesis after local electron irradiation appear much earlier than after irradiation with X- and gamma-rays.
The decrease in the number of Bcl-2-positive cells induced by electron irradiation is associated with the inhibition of translation of Mcl-1 mRNA (a protein related to Bcl-2) and its rapid destruction due to proteasome degradation mediated by phosphodegron with the participation of the GSK-3 signaling pathway, which leads to shift of the proliferative-apoptotic balance towards apoptosis (16).
Modulation of the activity of the above cascades can lead to chromosomal mutations, which is confirmed by an increase in the proportion of Cas-3- and p53-positive germ cells. It should be noted that after irradiation, a decrease in the number of mitotically dividing generations was observed, while during normal spermatogenesis, the pool of meiotically dividing gametes mainly decreases due to their active selection during crossing over (17).
Urgent effects at the organ level include the appearance of azoospermia, and delayed effects include dystrophic changes, the mechanism of which is decomposition, that is, the breakdown of cellular ultrastructures, leading to the accumulation of an excess amount of protein or lipids in the cell.
The morphological feature of electron irradiation in the testes is the absence of a complex, complex local vascular-mesenchymal reaction to damage to the germinal epithelium and seminiferous convoluted tubules, as well as their microenvironment, caused by the action of the studied ionizing radiation.
Conclusions
In the experimental model, local irradiation of the testes with electrons at a dose of 2 Gy causes the development of focal hypospermatogenesis, affecting up to 1/8 of the sections of the tubules (on the 1st week) with a subsequent increase to 1/4 (on the 5th week), with a tendency to recovery on the 11th week (temporary azoospermia).
The basis of focal hypospermatogenesis is the irradiation-induced proliferative-apoptotic imbalance towards the dominance of apoptosis, which is most pronounced in the pool of spermatogonia, with a sharp increase in the number of TUNEL-positive germ cells.
Acknowledgments
The author expresses his gratitude to the professor and reproductologist Borovaya T.G., MD, PhD; professor of N.F. Gamaleya Federal Research Center for Epidemiology and Microbiology; to Andreeva Yu.Yu., MD, PhD, professor of Russian Medical Academy of Continuous Professional Education, a specialist in the male reproductive system and to Koryakin S.A., PhD, Head of the Department of Biophysics of National Medical Research Radiological Centre of the Ministry of Health of the Russian Federation for assistance in conducting this study.
Funding: None.
Footnote
Reporting Checklist: The author has completed the ARRIVE reporting checklist. Available at https://tau.amegroups.com/article/view/10.21037/tau-22-598/rc
Data Sharing Statement: Available at https://tau.amegroups.com/article/view/10.21037/tau-22-598/dss
Peer Review File: Available at https://tau.amegroups.com/article/view/10.21037/tau-22-598/prf
Conflicts of Interest: The author has completed the ICMJE uniform disclosure form (available at https://tau.amegroups.com/article/view/10.21037/tau-22-598/coif). The author has no conflicts of interest to declare.
Ethical Statement: The author is accountable for all aspects of the work in ensuring that questions related to the accuracy or integrity of any part of the work are appropriately investigated and resolved. All manipulations were carried out in accordance with the International Recommendations for Biomedical Research Using Animals (EEC, Strasbourg, 1985), the European Convention for the Protection of Vertebrate Animals used for Experimental or other Scientific Purposes (EEC, Strasbourg, 1986), and Guidelines for Medical -biological research on the care and use of laboratory animals (ILAR, DELS). Experiments were performed under a project license (No. 043 dated August 11, 2020) granted by institutional ethics committee of Sechenov University, in compliance with national and institutional guidelines for the care and use of animals.
Open Access Statement: This is an Open Access article distributed in accordance with the Creative Commons Attribution-NonCommercial-NoDerivs 4.0 International License (CC BY-NC-ND 4.0), which permits the non-commercial replication and distribution of the article with the strict proviso that no changes or edits are made and the original work is properly cited (including links to both the formal publication through the relevant DOI and the license). See: https://creativecommons.org/licenses/by-nc-nd/4.0/.
References
- Vander Borght M, Wyns C. Fertility and infertility: Definition and epidemiology. Clin Biochem 2018;62:2-10. [Crossref] [PubMed]
- Jafari H, Mirzaiinajmabadi K, Roudsari RL, et al. The factors affecting male infertility: A systematic review. Int J Reprod Biomed 2021;19:681-8. [Crossref] [PubMed]
- Qu N, Itoh M, Sakabe K. Effects of Chemotherapy and Radiotherapy on Spermatogenesis: The Role of Testicular Immunology. Int J Mol Sci 2019;20:957. [Crossref] [PubMed]
- Abd El Tawab AM, Shahin NN, AbdelMohsen MM. Protective effect of Satureja montana extract on cyclophosphamide-induced testicular injury in rats. Chem Biol Interact 2014;224:196-205. [Crossref] [PubMed]
- Meistrich ML. Effects of chemotherapy and radiotherapy on spermatogenesis in humans. Fertil Steril 2013;100:1180-6. [Crossref] [PubMed]
- Guo M, Hay BA. Cell proliferation and apoptosis. Curr Opin Cell Biol 1999;11:745-52. [Crossref] [PubMed]
- Zhao WP, Wang HW, Liu J, et al. Positive PCNA and Ki-67 Expression in the Testis Correlates with Spermatogenesis Dysfunction in Fluoride-Treated Rats. Biol Trace Elem Res 2018;186:489-97. [Crossref] [PubMed]
- Bagheri H, Salajegheh A, Javadi A, et al. Radioprotective Effects of Zinc and Selenium on Mice Spermatogenesis. J Biomed Phys Eng 2020;10:707-12. [PubMed]
- Reisz JA, Bansal N, Qian J, et al. Effects of ionizing radiation on biological molecules--mechanisms of damage and emerging methods of detection. Antioxid Redox Signal 2014;21:260-92. [Crossref] [PubMed]
- Galluzzi L, Vitale I, Aaronson SA, et al. Molecular mechanisms of cell death: recommendations of the Nomenclature Committee on Cell Death 2018. Cell Death Differ 2018;25:486-541. [Crossref] [PubMed]
- Demyashkin GA, Borovaya TG, Andreeva YY, et al. An experimental approach to comprehend the influence of platelet rich growth factors on spermatogenesis. Int J Radiat Biol 2022;98:1330-43. [Crossref] [PubMed]
- Demyashkin GA, Borovaya TG, Andreeva YY, et al. Influence of platelets growth factors on spermatogenesis after electronic irradiation. Arkh Patol 2022;84:20-8. [Crossref] [PubMed]
- Marzban M, Anjamshoa M, Jafari P, et al. Effects of gamma rays on rat testis tissue according to the morphological parameters and immunohistochemistry: radioprotective role of silymarin. Electron Physician 2017;9:4524-32. [Crossref] [PubMed]
- Topcu A, Mercantepe F, Rakici S, et al. An investigation of the effects of N-acetylcysteine on radiotherapy-induced testicular injury in rats. Naunyn Schmiedebergs Arch Pharmacol 2019;392:147-57. [Crossref] [PubMed]
- Hall EJ, Giaccia AJ. Radiobiology for the radiologist. 8th edition. Philadelphia, PA, USA: Wolters Kluwer, 2019.
- Morel C, Carlson SM, White FM, et al. Mcl-1 integrates the opposing actions of signaling pathways that mediate survival and apoptosis. Mol Cell Biol 2009;29:3845-52. [Crossref] [PubMed]
- Sinha Hikim AP, Lue Y, Diaz-Romero M, et al. Deciphering the pathways of germ cell apoptosis in the testis. J Steroid Biochem Mol Biol 2003;85:175-82. [Crossref] [PubMed]