Latest advancements in ureteral stent technology
Introduction
The upper urinary tract can become obstructed because of several physio-pathological conditions or diseases. The aetiology of obstruction can be intraluminal (i.e., due to renal or ureteral stones, ureteral strictures, or papillary urothelial neoplasms) or extramural (i.e., due to advanced urological or non-urological neoplasia). Ureteral blockages increase the ureteric backpressure and—if left untreated—can result in kidney failure. In 1960s, catheters made of silicone rubber were introduced as the first generation of ureteral stents and employed as a temporary measure to by-pass ureteric blockages. Since then, the stent technology has undergone many developments, encompassing the constitutive material, surface coating, and design of the stent. Currently, a typical stent architecture consists of a 22–24 cm long flexible tube made of polymeric or metallic materials, with side-holes punched alongside its body. The two extremities of the stent are J-shaped (also known as pig-tail ends) and are designed to anchor the stent in the renal pelvis and bladder, thus preventing it from migrating (1) (see Figure 1).
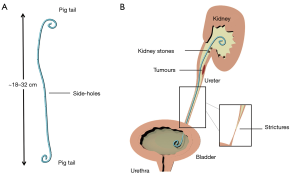
Over 1.5 million ureteral stents are used every year worldwide; however, it is estimated that >80% suffer from failure, which can cause severe pain and negatively impact on a patient’s quality of life, may require surgical re-intervention, and ultimately increase the healthcare economic burden (2,3). Some of the underlying causes of failure are ureterovesical reflux, tissue irritation, and formation of infectious crystalline biofilms (4,5).
Over the last few years, a large body of research has focused on innovating the stent technology, predominately through the development of materials and architectural features that may prevent or delay stent-associated complications. These have been discussed in recent review articles (6,7). In the present review, we highlight the very recent advancements in stent technology.
Advances in the constitutive materials or surface coatings
Several composite materials have been investigated for application in endourological devices (6,8); however, their usage has not led to a significant reduction in the incidence of stent encrustation or biofilm formation (9). The following section highlights recent advances in the constitutive materials of the stent, which are also summarised in Table 1.
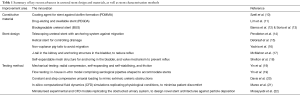
Full table
Szell et al. (10) developed a coating agent to prevent biofilm formation on stents, in the form of a poly(N,N-dimethylacrylamide) (PDMMA) hydrogel with antifouling and protein-repellent properties. In their study, bacterial proliferation and adhesion were evaluated in-vitro. The hydrogel layer was deposited on both polyurethane (PU) and cyclin olefin polymer (COP) glass slides, which were incubated in sterile human urine for 48 h. Uropathogens were then added to the medium and, after further incubation (24 to 48 h), bacterial proliferation was quantified by CFU counting. PDMAA coating on both PU and COP surfaces significantly decreased (5-fold) the presence of bacteria adhered on the surface.
Some commercially available stents (e.g., Universa® Soft Ureteral Stent; COOK® Medical, Bloomington, IN, USA) are coated with hydrophilic hydrogels (usually PVA) that become lubricious and reduce surface friction upon deployment. Hydrogels can also be utilised as a substrate to achieve controlled release of biologically active compounds. For instance, Lim et al. (11) developed a drug-eluting ureteric stent, allowing for a sustained drug release over time (up to 4–6 weeks, in-vitro) which improves drug absorption by the urothelium. The stent is spray-coated with a blend of a biodegradable polymer (70/30 poly-L-lactide-co-caprolactone, PLC) and an anti-proliferative drug (mitomycin C, MMC). This layer is then coated with polyethylene glycol diacrylate (PEGDA) hydrogel. PEGDA is a swellable polymer, thus the size of this outer coating layer increases once the stent is deployed, reducing the gap between stent and urothelium (~1.5 mm). Moreover, the hydrogel layer prevents the drug from being rapidly washed away by the urine flow, and retains it in proximity to the stent. Such a stent could be potentially used for the treatment of diseases affecting the urothelium, such as tumours or strictures. A pilot in-vivo study in a porcine model also demonstrated that the stent inserts easily in the upper tract, does not damage the urothelium or compromise kidney function, and does not cause hydronephrosis or systemic toxicity.
One aspect of significant interest in the last year has been the evaluation of biodegradable ureteral stents (BUS) using porcine animal models in-vivo. Biodegradable stents present several benefits, such as decreased patient discomfort and anxiety, and reduction of healthcare costs (including those associated with the “forgotten stent syndrome”). They are also particularly suitable for paediatric patients, to avoid anaesthesia during removal of the stent. BUS have been recently manufactured by Barros et al. (12), using an aqueous solution of gelatin-alginic-acid sodium salt and bismuth carbonate basic. Soria et al. (13) instead used a copolymer (Glycomer 631) and a polymer (polyglycolic acid). In both studies, BUS degradation took place in a controlled and predictable fashion, and no obstructive fragments appeared. Despite additional experiments are required to further validate this technology, it is evident that biodegradable stents represent an important avenue in future stent technology.
Advances in stent design
The development of novel stent designs has recently focused on stent architectures that could reduce tissue irritation and urinary reflux. The following section highlights recent advances in stent design (and their background claims), most of which have been patented. A summary of these inventions is also provided in Table 1.
Pendleton et al. (14) introduced a telescoping ureteral stent structure with the aim of improving stent patency; this design has been translated into a commercial device by COOK® Medical. It comprises a distal structure (located towards the kidney) telescopically sliding into a proximal structure (located towards the bladder). The invention also involves a number of new anchoring mechanisms that would act in two ways, (I) by stopping the stent from migrating inside the ureter and (II) by preventing the extended proximal end from returning back into the ureter. Additionally, novel deployment methods have been proposed for these stent models.
DeGraaf et al. (Boston Scientific Corporation, Grove, MN, USA) (15) introduced an helical stent made of polymeric materials, with the aim of controlling urine drainage through the stent. The embodiments of this invention consist of filaments with controlled extension properties, which are coiled around the stent lumen, together with a dissolvable coating. The degree of stent extension is defined based on the material stiffness, filament dimension, filament shape, and the method of extension.
Yachia et al. (Innoventions Ltd., Akiva, Israel) (16) invented a stent design that aims to reduce patient’s discomfort and flank pain. It relies on having both pig-tail ends non-coplanar with respect to the bladder trigone. The proximal end consists of a sleeve made of a softer material, which allows controlling the expansion of the pig tail depending on the level of urine force compared to the sleeve radial tensile force. Additionally, they introduced different shapes of the proximal region with the aim of preventing urine reflux from the bladder towards the kidney, due to increased bladder pressure. Another design element is a pre-shaped wire (made of polymer or metal) that is inserted through a small lumen parallel to the main stent lumen, with the purpose of retaining the curly shape of the stent.
McMahon et al. (Baylor University, Texas, USA) (17) introduced a stent design with potential for reducing ureterovesical reflux. It includes a distal J-end (kidney) and a novel anchoring structure at the proximal end (bladder). The lumen is designed to have a narrower cross-section in the bladder, in order to reduce bladder irritation. This segment of the stent could be expanded or folded depending on the level of anchoring required. Moreover, a flapper valve was designed at the bladder end, which closes when the bladder pressure increases in order to prevent reflux.
Shelton et al. (Gyrus ACMI®, Massachusetts, USA) (18) introduced a series of ureteral stent designs, with the aim of reducing urothelial tissue irritation, particularly at the bladder trigone and the uretero-vesical junction (UVJ). This design concept relies on projections (with different shape, length, size, and orientation), a self-expandable mesh structure anchoring in proximity to the UVJ, and coiled tails which may have a different internal diameter compared to the ureteric section of the stent. The invention also includes the integration with a valving mechanism to prevent reflux.
Pre-clinical testing of stent function
Yin et al. (19) introduced a novel stent manufacturing technology based on freeze-casting, in order to generate porous stents with improved urine drainage. In their study, stents manufactured with this technique were compared to standard 8 Fr double-J stent (Universa® Soft Ureteral Stent with Hydrophilic Coating, Cook® Medical, Bloomington, IN, USA). To understand the surface structure, both types of stent were imaged by scanning electron microscopy (SEM) and confocal microscopy, to characterise transverse and longitudinal sections, and both inner and outer surfaces of the stent. Mechanical testing was performed, including radial compression, self-expanding and self-stabilising testing, and friction testing (to determine the friction force due to a known displacement rate of 0.01 mm/s). The flow performance of the stent was investigated using an in-vitro model developed in house, comprising serological pipettes that were shaped to accommodate a stent. Results from these tests showed that the porous stent had improved drainage compared to the standard one.
Davis et al. (20) developed a method to investigate the ability of stents to resist extrinsic ureteric obstructions. It relies on the application of a constant or step compressive uniaxial loading in a direction orthogonal to the stent axis, at three different locations (proximal, central, and distal). Two types of load were investigated which were applied through (I) a 625 mm2 square surface simulating a large extrinsic obstruction, and (II) a metal rod (1 mm radius) to simulate a confined obstruction.
Marzo et al. (21) introduced an efficient urinary drainage test system, specifically designed for urethral catheters. They employed theoretical analytical methods, computational fluid dynamics (CFD) simulations and a standard experimental design to investigate the effect of catheter diameter on urinary drainage, with the ultimate goal of identifying a catheter design that could reduce patient discomfort. Their theoretical model was designed to replicate the physiological properties of the urinary system, specifically bladder and urethral region (geometry and flow dynamics of the urine flow through a bent tube). Results show that reducing the catheter inner diameter by half of its original size would significantly improve clinical tolerability while maintaining an acceptable flow rate. Moreover, the CFD simulation package allowed investigating the effect of catheter design changes on the spatial distribution of wall shear stress (WSS) and urine velocity.
The utility of CFD modelling as a tool to innovate stent design is also evident in the work by Mosayyebi et al. (23). In their earlier study (22), they developed a microfluidic platform (known as ‘stent-on-chip’) to investigate the mechanism of particle accumulation in ureteric stents. Using this model, they demonstrated an inverse correlation between the magnitude of shear stress acting on the stent surface (computed from CFD simulations) and the accumulation of encrusting particles. Moreover, they identified regions of the stent that are more likely to suffer from encrustation, such as inactive side-holes and other stagnant regions in the vicinity of a ureteric obstruction. Results qualitatively agreed with observations on stents retrieved from patients. Building upon this study, the same group investigated changes to the stent geometry, by varying the stent wall thickness and the shape of side-holes. They concluded that a thinner stent with streamlined side holes offers a 90% reduction in particle deposition compared to a standard stent design.
Conclusions
The present manuscript reviews recent developments in stent technology, with a focus on stent material, design, and characterisation methods. The most notable advances in stent materials include antibacterial and drug-eluting coatings, and biodegradable stents. Innovations in the stent design focused on reducing ureterovesical reflux, stent migration, and tissue irritation.
Despite significant efforts have been devoted to the improvement of current stent technologies, an ideal stent that does not suffer from failure and complications does not yet exist. However, we anticipate that this could be achieved through simultaneous developments of multiple technological features and properties of a stent, and by establishing appropriate computational and experimental design optimisation and verification procedures.
Acknowledgments
None.
Footnote
Conflicts of Interest: The authors have no conflicts of interest to declare.
Ethical Statement: The authors are accountable for all aspects of the work in ensuring that questions related to the accuracy or integrity of any part of the work are appropriately investigated and resolved.
References
- Albala DM, Gomella LG, Morey AF, et al. Oxford American handbook of urology. Oxford University Press; 2010:571-658.
- Davenport K, Kumar V, Collins J, et al. New Ureteral Stent Design Does Not Improve Patient Quality of Life: A Randomized, Controlled Trial. J Urol 2011;185:175-8. [Crossref] [PubMed]
- Harber M. Practical Nephrology. Springer; 2014:439-52.
- Urinary Stone Disease: The Practical Guide to Medical and Surgical Management (Current Clinical Urology). 2007 ed. Current Clinical Urology. Humana Press; 2007.
- Keane PF, Bonner MC, Johnston SR, et al. Characterisation of biofilm and encrustation on ureteral stents in-vivo. Br J Urol 1994;73:687-91. [Crossref] [PubMed]
- Mosayyebi A, Manes C, Carugo D, et al. Advances in Ureteral Stent Design and Materials. Curr Urol Rep 2018;19:35. [Crossref] [PubMed]
- Chew BH, Lange D. Advances in ureteral stent development. Curr Opin Urol 2016;26:277-82. [Crossref] [PubMed]
- Mosayyebi A, Vijayakumar A, Yue QY, et al. Engineering solutions to ureteral stents: material, coating and design. Cent European J Urol 2017;70:270. [PubMed]
- Cauda V, Chiodoni A, Laurenti M, et al. Ureteral double-J stents performances toward encrustation after long-term indwelling in a dynamic in vitro model. J Biomed Mater Res B Appl Biomater 2017;105:2244-53. [Crossref] [PubMed]
- Szell T, Dressler FF, Goelz H, et al. In Vitro Effects of a Novel Coating Agent on Bacterial Biofilm Development on Ureteral Stents. J Endourol 2019;33:225-31. [Crossref] [PubMed]
- Lim WS, Chen K, Chong TW, et al. A bilayer swellable drug-eluting ureteric stent: Localized drug delivery to treat urothelial diseases. Biomaterials 2018;165:25-38. [Crossref] [PubMed]
- Barros AA, Oliveira C, Ribeiro AJ, et al. In vivo assessment of a novel biodegradable ureteral stent. World J Urol 2018;36:277-83. [Crossref] [PubMed]
- Soria F, Morcillo E, Serrano A, et al. Evaluation of a new design of antireflux-biodegradable ureteral stent in animal model. Urology 2018;115:59-64. [Crossref] [PubMed]
- Pendleton SL, Neff GL, Biltz BT. Telescoping ureteral stent. Google Patents, 2018.
- DeGraaf K, Harrah TP, Boden MW, et al. Controlled extension stent. Google Patents, 2018.
- Yachia D, Ponomarenko V. Stent and Method of Use. Google Patents, 2018.
- McMahon CW, Nief CA, Schmidt DR, et al. Ureteral stent and method. Google Patents, 2018.
- Shelton KG, Prats AE. Ureteral stent with anti-migration features. Google Patents, 2018.
- Yin K, Divakar P, Wegst UG. Freeze-casting porous chitosan ureteral stents for improved drainage. Acta Biomater 2019;84:231-41. [Crossref] [PubMed]
- Davis NF, Mulvihill JJE, Lynch JJ, et al. Digital and Mechanical Characterization of Ureteral Stent Luminal Reduction in Response to Extrinsic Compression Forces. J Endourol 2018;32:1148-53. [Crossref] [PubMed]
- Marzo A, Melis A, Unger J, et al. An engineering approach towards a more discrete and efficient urinary drainage system. Proc Inst Mech Eng H 2019;233:58-67. [Crossref] [PubMed]
- Mosayyebi A, Yue QY, Somani BK, et al. Particle Accumulation in Ureteral Stents Is Governed by Fluid Dynamics: In Vitro Study Using a "Stent-on-Chip" Model. J Endourol 2018;32:639-46.
- Mosayyebi A, Lange D, Yann Yue Q, et al. Reducing deposition of encrustation in ureteric stents by changing the stent architecture: A microfluidic-based investigation. Biomicrofluidics 2019;13:014101. [Crossref] [PubMed]